Viruses: The Zombies Among Us
In the world of tiny things, nothing is smaller or more devastating than viruses that we cannot see with the naked eye but that hold the potential to kill millions and bring the world economy to a halt. Viruses, like zombies, are not living, so they can’t be killed. Many different infectious agents, such as bacteria, worms, and fungi are living organisms and can be killed. Bacteria, for instance, can be killed by antibiotics. Living organisms have the ability to acquire nutrients from their surroundings as allowing them to grow into their full form and become able to produce descendants and thereby maintain their population, or better, increase their population. Viruses are unique in that they can not physically move by themselves and are not able to use nutrients to make new viruses by themselves. However, they are also very durable: a viral particle can be frozen or kept in a vacuum without damage. Only high heat or high acidity will normally destroy viruses before they enter a human body.
Why Are There Viruses? What Are They Good For?
Viruses are not necessary for any biological purpose. Life for all organisms would be better without them. In contrast, while some bacteria can cause serious diseases, most bacteria are harmless to humans, and many hundreds of bacterial species live on our skin, or within the intestines of humans, and provide a biologically important function. We benefit from their functions in maintaining proper digestion and health.
Viruses originated as “unused parts” or “spare parts” from the process whereby bacteria undergo cell duplication. This duplication process is normally sufficiently effective to produce trillions of bacteria. But, it is not perfect, and sometimes a bacterial cell division may not be completed, leaving parts behind from an unfinished new bacterial cell. Most of the time these unfinished parts are simply degraded, either by some nearby living bacteria, or by our human lymphocytes (if this occurs inside our bodies). Lymphocytes are the protective cells of our immune system and include macrophages (Greek for “big eaters”) that devour foreign cells or viruses.
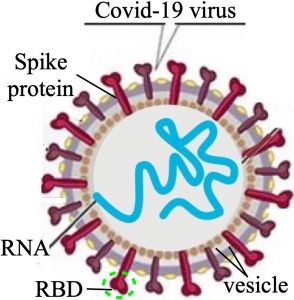
Figure 1. A SARS CoV-2 virus. RBD = Receptor Binding Domain; it binds to the ACE2 receptor in Fig. 2.
But, occasionally some of these spare parts, if they contain fragments of the original bacterial DNA or messenger RNA, can become encapsulated in a vesicle (a small sac formed by membranes; see fig. 1). Being enclosed by this vesicle allows viruses to resist being degraded. Even less frequently, such a vesicle may acquire a surface protein, such as the spike protein (see fig. 1), by which it can now bind to a surface protein on a bacterial cell, or on a human cell (see figs. 2 and 3). When this happens, a meaningless spare part becomes an invading infectious agent.
Such new viral particles will be “successful” for the virus if the fragments of genetic information (the RNA in fig. 1) happen to contain just the information for reproducing the viral molecules into another identical copy of this virus. Therefore, the only purpose of a virus is to infect a cell, where it forces the cell to use the established cellular machinery that cells use for their own functions, that is, to make thousands of copies of the invading viral particle. To summarize: a virus simply contains the genetic instructions for making more viruses, and because it can invade living cells, it can then be produced in very large numbers to be spread by blood to other cells inside our body. If the infected person coughs or sneezes the virus can also spread by air, or by water to other organisms. Because viral infection normally results in the infected cells dying, this is therefore quite harmful to the infected organism, such as humans.
How Do Viruses Invade Us?
Viruses are remarkably efficient infectious agents. There are about 900 different viruses that infect at least one or more animal species. For the virus to cause an infection, it must first enter the cells of the host organism. This infection process depends on at least two separate features: the host cell must have at least one specific cell-surface protein (one that sticks up or out of the cell; see fig. 2), and the virus must have at least one viral surface protein (see fig. 1) that uniquely recognizes this host cell protein and binds to it (see fig. 2).
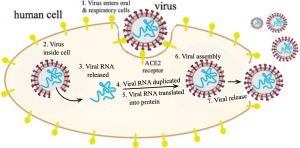
Fig. 2. The route by which the virus enters a human cell, where many copies of the virus will be made, and released, to spread the infection to other hosts. ACE2 = Angiotensin Converting Enzyme #2.
For the COVID infection, the human protein is ACE2 or Angiotensin Converting Enzyme (ACE2 in fig. 2), a protein found on the membrane of cells lining blood vessels, and also on epithelial cells, which line the oral cavity and the lungs. Its normal function is in maintaining blood pressure by binding angiotensinogen (a hormone precursor) and converting it to angiotensin, the active hormone for controlling blood vessel expansion (vasodilation) or narrowing (vasoconstriction).
If one inhales air from an infected person who coughs or sneezes, the virus can enter any epithelial cell in our oral cavity or lungs. Once inside these cells, the virus can then cross into an adjacent capillary. Because our smallest blood capillaries reach every part of our body, once inside our blood COVID viruses can reach every possible cell that would be served by any capillary. Thus, a spike protein that can recognize and bind to an ACE2 protein was a very fortuitous chance event that has made the COVID particle such a successful virus for invading all our cells.
By this binding interaction the virus is then pulled into the membrane of the host cell (fig. 2, steps 1 and 2) so as to commandeer the cell’s normal machinery for making RNA and proteins, whereby the host cell now makes many thousands of copies of the invading virus (steps 3–6). The cell soon dies, causing it to break up, releasing these viruses to travel via the blood to other organs/tissues (step 7), thereby increasing this infection enormously within only four to seven days. Because these receptor proteins on cell surfaces differ enough from one species to another, most viruses normally can infect only one animal species. But—sometimes chance leads to a mutation in the viral surface protein, so that it now can bind to a slightly different type of animal cell receptor. This has occurred many times with different viruses, so that a virus that originated in a bat or a bird then moved to a pig or a duck, and because people have more contact with domestic animals, the virus can then mutate again and easily contact and infect humans.
COVID-19
An unfortunate example of this is the virus causing the current pandemic. Two different names are associated with this plague and have slightly different meanings. The actual disease is normally referred to as COVID-19 (Corona Virus Disease 2019).
The infectious agent itself—the virus—belongs to a viral family noted for its surface pattern of spike proteins that gives the appearance of a crown and hence is defined as a corona virus (see fig. 1). This virus is quite effective at entering the human lung where it causes rapid lung cell death, leading to Severe Acute Respiratory Syndrome or SARS. Because the type that is so harmful to humans is the second member of this Corona Virus class, its full name is SARS CoV-2.
How Bad Is COVID-19?
At the end of spring 2021, it is apparent that COVID-19 will be the deadliest event in American history. Table 1, with data for flus from the Center for Disease Control, permits direct comparisons with other major disasters. Only the Civil War, where the dead on both sides were Americans, and at a time when medical assistance for the wounded was still quite limited, is comparable to the death toll for a flu epidemic. By the end of May 2021, the CDC reported over 3.3 million deaths due to COVID-19 on our planet.
But people ask: how likely am I to get COVID and possibly die? For the general population, one is 15 times more likely to die after infection with SARS-CoV-2 compared to the seasonal flu. The likelihood of infection leading to death is influenced by the general health of an infected person. Older people often have poorer health or weaker immune systems. And all people who are obese or suffer any other chronic ailments have death rates at least double those of healthy people who become infected.
How Do We Defend Ourselves Against the Virus?
Vaccines are essential to prevent viral diseases because viruses cannot be removed by antibiotics. To help protect us against any possible infectious agent, whether a whole organism or only a foreign protein, our immune system constantly makes an enormous diversity of different antibodies, so that there is a good chance that, whatever new invader enters our body, we will already have at least a few antibody molecules that are uniquely shaped to bind to this infectious agent, thereby attracting macrophages that will consume and destroy the invading organism. Once macrophages begin to consume such antibody-tagged invaders, this sends a signal to make many more of these specific antibodies. It normally takes 4–7 days for the immune system to achieve full scale production, which is why for most of us a flu, or other infection, normally lasts 4–7 days, until it is successfully overcome.
Expected Future COVID Variants
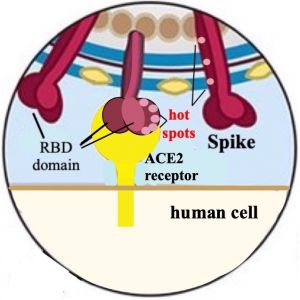
Figure 3. Predicted sites for mutations (hot spots) in the spike protein’s RBD and stem. Compare with Fig. 2.
By the end of spring 2021, we have already observed at least six important variants of the original COVID-19. Such variants arise when a chance mutation of an amino acid in the spike protein, especially if it occurs in the Receptor Binding Domain (RBD), can alter the ability of the antibodies, produced in response to a vaccine, to recognize these altered variants. In this arms race the virus is limited in what type, and how many changes (mutations) it can make, because if the RBD changes too much, it will no longer recognize a human ACE2, and the virus will be unable to enter a human cell, and cease to propagate. By studying the structures of both the spike protein and the human Angiotensin Receptor Enzyme (ACE2) scientists have been able to predict which amino acids in the viral spike protein are possible spots for a mutation that could help the virus evade antibody detection, while still being able to bind to ACE2. An example of such modeling is shown in fig. 3. Some mutations of COVID-19 have already occurred, making it more certain that with millions of infected people, such large numbers will give enough opportunities for other mutations to occur by chance, and then thrive because they can avoid immune detection. To help in understanding this, note that the RBD is at the end of the spike protein—the tip of the protein on the viral surface shown in fig. 3, and shown as binding to the human ACE2 receptor. This tip is what an antibody protein would most easily encounter within our blood if it comes near to a viral particle and is then the part of the spike protein that the antibody can recognize, and then bind to, so as to neutralize the virus.
Successful mutations may also occur in the stem of the spike protein (the region above the RBD in fig. 3), if these cause the stem to twist enough so as to change the orientation of the surface. Such a new orientation might make existing antibodies, against a previous version of COVID, no longer recognize the altered appearance. That would be enough for this mutation to also be successful for the virus and defeat our ability to destroy it.
The Delta Variant
Originating in India, late in 2020, this variant of COVID-19 has three new mutations. These mutations have increased the efficiency with which the virus attaches to, and then enters, ACE2-carrying cells. This has made this variant expand very rapidly so that it will be the dominant variant in much of the world by the summer of 2021. Vaccines against the classic COVID-19 are still helpful against this variant, but no longer as effective.
How Good Are Vaccines Against COVID?
Even with the delta variant being more infectious, and becoming the dominant strain, the CDC reported that for the month of May 2021, with over 18,000 deaths in the US from COVID, only 0.8% were in fully vaccinated people. A better way to state this: for people who died from COVID that month, more than 99% had not been vaccinated. Vaccines work.
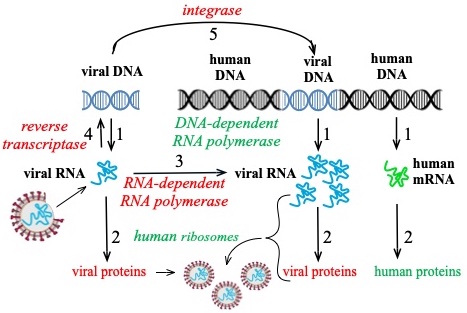
Fig. 4. Process for viral enzymes (red) to produce more viral RNA, or to insert new viral DNA into human DNA. Human enzymes in green.
Why Does the Virus Use RNA for Its Genome?
The cells of all mammals contain nuclei, and within these nuclei is contained the total genomic information, in the form of DNA, for making more than 10,000 different proteins. The DNA consists of two strands that form a double helix (fig. 4) and a single normal gene will be an average of 50 times larger than the messenger RNA that is copied from it, by human RNA polymerase (step 1). Ribosomes (step 2) then use this human mRNA as a template for making human proteins.
The single COVID RNA contains all the virus’ genomic information, similar to human DNA, and also acts as its own messenger, similar to human mRNA. It is then evident that the viral capsid—the spherical part surrounding the RNA in fig. 1—can be correspondingly smaller, and easier to construct when it only has to contain this RNA. COVID RNA also contains the information for an enzyme that will make more COVID RNA (step 3) to accelerate the ability to make viral proteins (step 2) needed to make many more viral capsids. A majority of viruses have an RNA genome because it can be very small, and this feature makes it easier for them to spread.
The virus needs only a few proteins to form the viral capsid, or shell, and it also has the blueprint in its own RNA that enables the invaded human cell to make the viral enzyme reverse transcriptase. This enzyme then makes a DNA version of the viral mRNA (step 4). From this viral DNA the cell’s RNA polymerase (step 1) can make many new copies of the viral mRNA, each of which will be inside a new viral capsid (fig. 2).
A more devious feature is that the virus also contains an integrase, an enzyme that can insert a piece of the new viral DNA somewhere into human DNA (step 5). With HIV (human immunodeficiency virus), this insertion of the viral DNA happens in almost all infected humans. A similar insertion of COVID DNA into human DNA has recently been observed by three science laboratories studying SARS CoV-2. With HIV patients there is no cure after this happens, and they are on antiviral medication for the rest of their life. How frequently COVID DNA becomes inserted into human DNA has not yet been determined.
Survival of the Fittest—By Being Much Less Efficient?
Since Charles Darwin developed the theory of evolution we have had thousands of examples of “survival by the fittest,” which we normally understand to mean the best. Polymerases are enzymes that form polymers of nucleotides to make either DNA or RNA. “Polymer” simply means a molecule with many components. They do this by sliding along a template, the DNA to be copied, and choosing the correct matching nucleotide at that same position in the new DNA or RNA being made. These enzymes are so good that they let the incorrect nucleotide slip in only once in every 20 million steps. Imagine a typist being that good. By comparison the reverse transcriptase for the HIV virus makes one error in every 2,000–4,000 steps, or about 3 million times worse! Every time an HIV virus’ RNA is duplicated to DNA, an average of 2 mutations occur. When the human RNA polymerase then makes RNA from that new viral DNA (fig.4, step 1), the mutations are passed along, and one or two of the proteins now made from that viral RNA will have a mutation. All of this has been verified many times with HIV, but as yet we do not have such detailed results with the COVID system. But since COVID viral RNA codes for the enzymes reverse transcriptase and integrase (fig. 4) it may well have comparable inefficiency, consistent with the emergence of so many new variants in only one year.
For the virus the clear benefit is that many copies are always being made, and if some have a bad mutation, they will not survive, but most viruses will continue. But in a world with vaccines, if a mutation allows the virus to escape detection by antibodies against an earlier version of the virus, then the new variant virus and its descendant will become the dominant strain and continue. The sloppy virus lives forever!
In Conclusion—What Can You Do?
First: Get vaccinated in order to destroy the virus as it enters your body, before it can use you to make many copies of itself, and jump by means of a sneeze, cough, or handshake to a new host. Second: Even after you are vaccinated, wear masks around unvaccinated people because there is the off chance that they can contaminate you and, even though you will not get sick, make you an unwitting spreader. Third: In short, the main goal for all of us is to prevent these real-life zombies, which viruses are, from using us as their fodder.
I have deep appreciation for this article, so clearly and comprehensibly written for those of us in non-scientific fields. I now understand the nature of viruses much better and therefore the means by which we all can counter by our own behaviors the spread of Covid-19 and other deadly viruses. Thank you for publishing this excellent and critically informative article.
Lucid and lively, this article clarified three key enzymes in the current pandemic. 1)The virus commandeers human cells to make reverse transcriptase to make viral mRNA.
2) Then, human cell’s polymerase manufactures by mass production viral proteins.
3)The viral integrase inserts COVID DNA into human DNA. The typographical errors during transcription makes mutations inevitable.
Zombies, indeed!
Thanks, Tom for this in depth look at this virus, explained in a way I could understand.
A great teacher can take complex issues, and inform others in a less complex way.
Thank you Tom. This is very helpful for the average person to get and idea of this terrible time. You have put a lot of work into this study
Thank you, Tom! You have taken many concepts and terms we may have heard over the years and integrated and explained their role regarding covid-19. This is extremely helpful to understand as we move forward in our current struggle.